This molecule is vital to our survival. Now we know what it looks like
In order to live, all mammals depend on the function of a large molecule in our nerve cells made up of four proteins. But what these proteins look like has been mostly unknown, making it difficult to treat diseases related to the molecule. Now, an international team of researchers, including a group from the University of Copenhagen, have mapped the missing pieces.
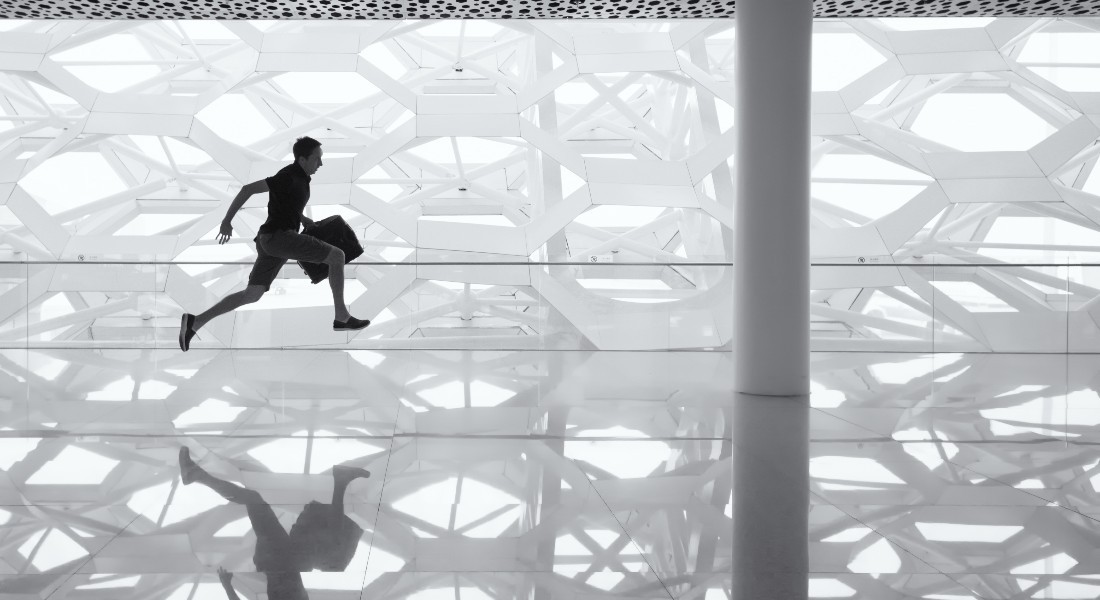
All movement, sleep, thinking and feeling depends on tiny electrical currents in your body. A large molecule in our nerve cells made up of four proteins controls these currents. Mutations interfering with any of the proteins in this molecule can severely disrupt your ability to move or sleep, or even cause life-threatening disease.
In fact, there are three syndromes associated with the malfunction of these proteins, but none of them can currently be treated. This is partly because we only knew the structure of two of the four proteins involved in the molecule.
That could now change based on a new collaborative study by researchers from the University of Copenhagen (UCPH), Genentech Inc, ETH Zürich and Columbia University. The study, which was published in Nature, maps the 3D structure of the two remaining proteins.
“The discovery enables us to better understand the function and structure of the entire molecule, and possibly how the proteins can be targeted to eventually treat patients,” says co-author Professor Stephan Pless from the Department of Drug Design and Pharmacology at the UCPH.
It has been clear to scientists for years that this massive molecule is required for survival of mammals. Even small mutations in the proteins of the molecule can have devastating consequences, and some humans carrying mutations in them do not survive childhood.
“While recent progress in AI-driven biology has hugely advanced our understanding of the 3D structures of many proteins in our bodies, this computational approach works less well for the very largest proteins in our body - including the two proteins we were missing the structures of in this assembly. Now we finally know what they look like in 3D, and we started to test and rationalize how human mutations affect its function,” explains Stephan Pless.
A global scientific collaboration
The first proteins in this molecule were discovered in 1987, but it was only just recently discovered that a minimum of four proteins are required for the molecule to function. The discovery was made by Dr. Han Chua Chow, Assistant Professor at the UCPH.
A team at Genentech, together with the Pless Group at UCPH, had determined the 3D structure and the function of two of the proteins in 2020, but the structure of the two largest proteins of the molecule remained unknown.
By dividing the task between the four research groups in the USA, Switzerland and Denmark, the researchers were now able to determine the structure and function of the two missing proteins.
The team at Genentech in San Francisco was able to obtain the first-ever 3D images of the two remaining proteins.
“Researchers at the ETH Zürich then broke up and analysed the molecule fragments to confirm the overall structure prediction. Another group at Columbia University in New York did single-molecule analysis, including on patient mutations that cause severe disease,” Stephan Pless explains.
With the studies from San Francisco, Zürich and New York, the team in Copenhagen put together the information and did an analysis of the function of the molecule.
“The 3D images confirmed functional data we had obtained some years ago and also gave us a first hint on how to maybe alter the function of the complex. In fact, we were able to design small protein fragments that disrupted the function of the molecule, which may be useful in the future because the complex is often hyperactive in patients,” says Stephan Pless.
A blind passenger
However, the researchers also discovered a fifth protein, a sort of blind passenger, that was clinging to the complex.
“Given the sheer size of this massive molecule, we had already suspected that there may be other proteins that might also interact with and possibly affect the function of the complex,” says Stephan Pless.
The fifth protein, calmodulin, is already very well described, but no one knew that it had anything to do with this molecule.
“When we purified the protein complex, this fifth protein was clinging onto it and would not let go. It tells us that it could have an important function for the overall complex. It also reveals that there might be other proteins than the ones we found, whose function may be important but still unknown to us. So, although we are very excited to map the overall complex, there are still many things that we cannot explain,” says Stephan Pless.
Read the full study ‘Structural architecture of the human NALCN channelosome’ in Nature here.
Contact
Professor Stephan Pless
stephan.pless@sund.ku.dk
+45 23 64 90 66
Press Officer Søren Thiesen
s.thiesen@sund.ku.dk
+45 28 75 29 34